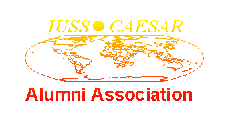
Exploiting "Conventional," Differential and Rotational Doppler
By Bruce Rule - Aug 15, 2014
INTRODUCTION
Doppler effect analysis can be appled to any measurable signal from very low frequency acoustic energy to well beyond the visible light spectrum to determine the velocity of an energy-radiating object relative to the observer's instrument (sensor) at ranges from feet to at least hundreds of millions of lightyears. (A lightyear - the distance light travels in one year - is about six trillion miles.) The more accurate the measurement of Doppler-induced frequency changes, the more accurate the derive velocity.
"CONVENTIONAL" DOPPLER
For the purposes of this article, the term "conventional" Doppler is applied to detections made by a single sensor (or array) over time of a stable signal to determine frequency changes produced by the motion of the source relative to that stationary sensor. From a "System" perspective, the most common application involves detection events during which the aspect of the source relative to the sensor changes from bow to stern, an evolution that, depending on range, can require extended periods of time.
A relationship that can be scaled for different frequencies and velocities to provide usefully accurate solutions or estimates is: a speed of 10 knots produces a full bow to full stern frequency change of 0.69 Hz at 100 Hz for a sound velocity of 4850 feet/second.
If the extent to which the source does not provide a full bow-to-stern aspect change can be accurately determined, the full bow-to-stern frequent change value can be derived (reconstructed) by applying (correcting for) the cosine of the angle of the "missing" bearing sector.
Example: if initial detection (and source measurement) is made at a reconstructed angle of 20 degrees off bow and the source (target) is subsequently delected at full-stern aspect (maximum down Doppler), and a frequency change of 1.5 Hz is measured for a 100 Hz source, then application of the cosine of the missing 20 degrees to the measured shift value of 1.5 Hz, the reconstructed full bow-to-stern Doppler shift at 100 Hz would be 1.75 Hz for a speed of 25.4 knots (1.75/0.69). The linked site below provides a cosine table.
Note: fn its most refined application, Doppler-derived speed solutions were established more than 30 years ago to be more accurate than own ship's log. During a Navy-sponsored evaluation of the technique that involved "target services," "12 of 13 (data point) solutions were found to have a standard percent of error of plue/minus one-half of one percent."
DIFFERENTIAL OR "INSTANTANEOUS" DOPPLER
Differential Doppler can be considered a sub-set of time-difference fixing. If a source is detected by three or more sensors that subtend more than 220-240 degrees of arc relative to the position of the source, measurement of the same acoustic source detected by these sensors at the same time on the target (corrected for sound travel time), a comparison of these values can provide target course and speed. As previously discussed, the more accurate (refined) the measurement input, the more accurate the solution. The advantage of this technique is that it does not require a continually stable acoustic source. It requires only that frequency measurements be made of the same source at the same "target time."
Programs that provide such solutions using only frequency inputs and the positions of the sensors have been in existence for more than 20 years. They have been described as "Rotating Field Solutions."
In special cases when simultaneously (same target time) detecting sensors are located nearly dead ahead (within less than five degrees of full bow aspect) and nearly dead astern (within less than five degrees of full stern aspect), only those two sensors are required to derive an accurate speed solution. Such measurements can be made independent of range.
ROTATIONAL DOPPLER
Astronomy provides a useful example of rotational Doppler. If the flattened disk of a rotating spiral galaxy is viewed nearly edge-on, measurements of the frequencies produced at known 'at rest" values by the excitation of specific elements in the stars located at the edge of approaching side of the galaxy (up-Doppler, blue-shifted light) compared to frequencies produced by the same elements in stars at the edge of the receding (down-Doppler. red-shifted light) side of the galaxy provide the rotational velocity of the galaxy.
If the galaxy is viewed from either directly above or directly below (along the axis of rotation extended), there is no rotational Doppler; the same frequency values are produced by specific stellar elements around the entire circumference (outer edge) of the galaxy, i.e., the entire galaxy presents a "beam aspect" (zero Doppler from rotation).
Detection of rotational Doppler from an acoustic source requires that the source have rotational velocity that exceeds the velocity of the "central" shaft that transfers motion to the radiating source.
An example of such a relationship would be a "singing" propeller where areas of one or more of the blades, which can be distant from the propeller hub and driving shaft by as much as five or more feet (for a large propeller) can be excited into resonances by flow-field forces that often exist only at critical ranges of speeds and/or depths.
If the speed of the propeller shaft of this example is 300 rpm (five revolutions per second: rps) and the area of a blade that is excited into a narrowband resonance (at 300 Hz, for example) is five feet from the center of the propeller shaft, the rotational velocity of that radiating surface will be 157 feet/second (10 X 3.141596 X 5 rps) or 93 knots.
As the area of the propeller blade that is "singing" revolves toward a sensor located directly abeam of the propeller, the 300 Hz source will be boosted by up-Doppler from 300 Hz to 309.6 Hz and will be lowered by down-Doppler to 290.4 Hz as the blade rotates away from the sensor.
So, exactly at CPA, (beam aspect) the 300 Hz source will have an apparent (detected) bandwidth of 19.3 Hz within the integration period of the signal processor if that integration period is greater than about half the period of the propeller rotation (0.1 seconds)
After the source (target) passes beyond CPA, the apparent bandwidth of the 300 Hz source will gradually decrease until it again becomes a narrowband signal at 300 Hz when dead-stern aspect is presented to the sensor, and there is no rotational Doppler component. The rate at which the bandwidth decreases is a function of the cosine of the changing angle between dead-beam and full astern.
What is the practical application of this esoteric analysis technique? Well, in one case, the writer was able to determine that a single blade of a controllable-pitch propeller was "singing" (resonating from flow-induced energy) at the point where that blade was attached to the pitch-control mechanism within the hub and not, as would have been expected, well out along the trailing edge of the blade. The blade was experiencing excessive vibration - essentially "chattering" - at the attachment point and was thus more likely to fail than the other blades.
Searches for rotational Doppler effects also offer potential insight into largely unknown areas involving the noise radiation mechanisms of rotating machinery. For example, how much of the apparent bandwidth of narrowband acoustic energy produced by mechanical imbalance forces acting on a rotating machine is the result of the signal being a combination of sources produced by different areas of the central shaft (important for extended shaft lines; see below) or by excitation of structures that support or are otherwise connected one-to-one to the shaft? Further, refined measurements of temporal changes in the detected bandwidth produced by rotational Doppler can refine the time that beam aspect is presented to the sensor even at significant detection ranges.
Bottom line: you never know what you will identify until you look, and it is highly probable no one is now looking for rotational Doppler effects. Any such analytical effort should also investigate the presence/absence of evidence of transient "differential rotation" - the probably unattainable "Holy Grail" of measurement - along extended shaft lines when they are heavily torque-loaded; such information would have implications for composition and structural integrity. The final step in such an effort should involve the use of A-scans to provide measurements of exquisite precision.
Far-fetched?- no more so than collateral assessments made in the l960s, 70s and 80s which were met with enormous skepticism but which are now accepted without comment as established facts.